1.IntroductionCervical cancer is the leading cause of mortality in female cancer and the second most common cancer in females worldwide.1 The introduction of new screening and treatment modalities has led to a decrease in mortality from cervical cancer over the last 50 years.2 3 However, in the last two decades nationwide screening programs have revealed an increase in the incidence of cervical cancer. Hornung et al.;4 estimated that mortality from cervical cancer will rise by 20 in the next decade unless improvements are made in current screening and detection techniques. Colposcopy has proved to be a useful diagnostic tool for identifying the most atypical site for biopsy of the cervix.5 6 When women with an abnormal Pap smear are referred for colposcopy, diagnosis and treatment of cervical intraepithelial neoplasia (CIN) requires several visits to the doctor. With conventional colposcopy, using acetic acid staining to select the most atypical site for taking a biopsy, only 53.6 of the biopsies removed contain histological evidence of a dysplastic process.6 In an overview of 14 papers made by Hopman et al.;, the positive predictive value of colposcopically directed biopsy was poor for “no CIN” and it increased with an increase in the stage of the disease.7 Also considerable interobserver variability with regard to diagnosing CIN is reported in the literature, for colposcopists as well as for histopathologists.8 9 10 11 Fluorescence imaging and spectroscopy are relatively new experimental techniques for the detection of superficial epithelial changes. Fluorescence diagnostics are based on detecting in vivo differences in fluorescence between normal and cancerous tissues. Fluorescence is induced by the excitation of fluorophores in the tissue, usually with blue or ultraviolet (UV) light. The area of interest is then imaged by a sensitive camera or a point measurement is performed by spectroscopy. The shallow penetration of the excitation light makes this tool particularly suitable for superficial lesions. Differences in fluorescence between normal and cancerous tissue can be present naturally due to different fluorescent molecules in normal and cancerous tissue or it may be due to different absorption or scattering in tissue.12 13 14 15 The use of natural tissue fluorescence spectroscopy for CIN screening was extensively evaluated and reviewed by Mitchell et al.; They concluded that fluorescence spectroscopy performs better than colposcopy.16 Differences in fluorescence between normal and cancerous tissue can also be enhanced by administration of a tumor localizing fluorescent drug that preferentially accumulates in cancerous cells. Several fluorescent tumor localizers are currently under study. 5-aminolevulinic acid (5ALA) is currently a popular drug for photodynamic therapy. When administered topically it diffuses into the cells and converts into the fluorescent Protoporphyrin IX (PpIX). Selective accumulation in certain cell types may be caused either by differences in the cellular enzyme level, or by differences in the accessibility to ALA.17 18 19 20 Hillemanns et al.; used this approach for CIN screening based on fluorescence imaging. They showed that fluorescence imaging for CIN screening has results similar to those of colposcopy. However by using a more quantitative method, fluorescence spectroscopy, with a fiber-optic probe at a fixed distance, they found a significant improvement over colposcopy.21 Sinaasappel and Sterenborg described a more quantitative method for fluorescence measurements, the double ratio (DR) measurement technique.22 Two excitation wavelengths ( λi and λj ) and two detection fluorescence wavelength ranges ( λm and λn ) are used to generate four different fluorescence images from which a DR image is calculated. The DR algorithm is designed to compensate for the color and light scattering of tissue and produces an image that is dependent on the amount of the fluorescent tumor localizer only:23 where Fx,y stands for the fluorescence obtained with excitation wavelength λx detected at wavelength λy and C stands for the concentration of the photosensitizer. The parameters α and C0 are unknown constants that are related to the fluorescence quantum yields of the autofluorophores and of PpIX. It can be shown23 that α for PpIX in tissue has a value of approximately 8. The DR is a smooth function of C/C0. At low values of C/C0 it is proportional to C/C0 and at higher values it saturates at a value of α. DR images therefore have a direct relation to the PpIX distribution of the imaged area, and are not biased by the usual optical artifacts.The aim of this clinical pilot study was threefold. First, we test our general purpose fluorescence imaging prototype in a clinical setting. Second, we evaluate the feasibility of using the ALA-DR approach as a fluorescence guide for taking biopsies. Third, we test whether this quantitative fluorescence imaging approach is capable of noninvasive staging of CIN. 2.Materials and Methods2.1.Patient SelectionWomen with an abnormal Pap smear, routinely referred to the clinic for colposcopic examination, were asked to participate in this study. Pregnant or lactating women were excluded. A total of 30 volunteers enrolled in this study. Their ages varied between 19 and 45 years with a mean age of 30 years. We excluded six volunteers with a history of previous surgery that had caused unknown changes to the epithelium. Another eight volunteers were colposcopically unsuspect for CIN and consequently no biopsies could be taken. Finally, 19 biopsies from 16 volunteers were available for our analysis. 2.2.Application of ALAThe ALA was purchased from Medac GmbH (Hamburg, Germany) in crystallized form. On the day of use it was dissolved in a concentration of 1 (wt/wt) in sterile saline solution with the pH adjusted to 5.5 using sodium hydrogen carbonate.21 Sterile gauzes drenched with 10 mL of this solution were applied to the cervix. The anticipated application time was 60 min, but in practice it ranged from 60 to 120 min. 2.3.DR Fluorescence Imaging PrototypeThe DR fluorescence camera we used is a general purpose prototype developed by our group. In practice it resembles an operating microscope. Preliminary clinical experiments were performed for neurosurgery and dermatology. The device uses two violet wavelengths for excitation of the cervix, 405 and 435 nm at intensities of about 100 and 200 μW/cm2, respectively. These are not the optimum wavelengths for DR imaging of PpIX, but it is a compromise between the contrast and signal to noise ratio. Excitation light is generated with a 200 W Hg–Xe lamp, two line filters, and a chopper that alternates the two excitation wavelengths. Fluorescence of the cervix is detected with an image intensified camera (Philips IP 800) through a red and a green filter (550 nm, bandwidth 42 nm and 675 nm, bandwidth 110 nm, respectively). In this manner four different fluorescence images are acquired. These are processed into DR images by ratioing the two red and green images and subsequently ratioing the two ratio images. A detailed description of this imaging device and the theory behind it can be found elsewhere.22 23 24 For focusing and positioning a simple white light (monochrome) imaging mode is available. 2.4.ProcedureAt the time of colposcopy the moist gauze containing the ALA is removed. Then a regular colposcopic procedure is performed, including acetic acid staining and photographic documentation of the cervix. In case the decision is made to take a biopsy, the location at which the biopsy will be taken is marked on the photograph (a Polaroid) that was taken to document the colposcopy procedure. Subsequently, the colposcope is removed and the fluorescence imaging device is positioned and focused. Positioning the device took 1–5 minutes, acquiring the fluorescence images took approximately 60 s. The resulting DR image was not available to the clinician during the procedure. Next, the fluorescence imaging device is removed and the colposcope is used to take the biopsy. The biopsy is then subjected to standard histopathological evaluation. 2.5.Data AnalysisThere were two blind steps in the procedure to avoid bias. First, as mentioned above, the clinician has not seen any fluorescence image before the biopsy is taken. Second, the results of the histopathologic evaluation of the biopsies were not available to us at the time of data processing. Defining the location of the biopsy site in the DR image was not simple since it involved matching two different images taken with different devices (i.e., the colposcope and the fluorescence imaging device). Without these two blind steps possible bias could have occurred. 3.Results3.1.GeneralAlthough the prototype functioned properly in the technical sense, its use in this particular clinical field proved to be very cumbersome. Positioning and focusing the device was especially difficult and time consuming. A typical example of a set of images is given in Figure 1(a). The speculum, visible on the right side of the white light image, often caused severe artifacts in the fluorescence image that were not easy to avoid. These artifacts were related to reflections of excitation and fluorescence light on the stainless steel surface that were not completely blocked by detection filters. In addition, the wall of the vagina (that part not covered by the speculum) was out of focus and usually fluoresced intensely. In the fluorescence image this area is painted black, so only the fluorescence on the cervix remains visible. Figure 1White light image and DR fluorescence images for two cases in which clinically no CIN was detected and consequently no biopsy was taken. (a) Normal cervix, fluorescence intensity is low, although some speckles are present. (b) Inflamed cervix. The DR values are close to 1, indicating relatively low PpIX levels. ![]() DR fluorescence images were taken of 16 patients, on whom a total of 19 biopsies were obtained and resulted in various different stages of CIN (Table 1). Table 1
3.2.DR Fluorescence Images of No CINFigure 1(a) shows the white light image and the corresponding DR image of the cervix of a patient who was colposcopically diagnosed as having no CIN. Although some speckles are visible, DR values close to 1 are found throughout the cervix, suggesting very minimal PpIX production. A similar situation is depicted in Figure 1(b), where the main difference is that this cervix is severely inflamed. Nevertheless, the DR image shows a uniform low value, suggesting low PpIX levels throughout the cervix. 3.3.Localization of CIN With DR Fluorescence ImagingIn four cases a region with clear boundaries could be recognized in the DR image. In all four cases this region matched the location where the biopsy was taken. The four lesions that showed localized fluorescence were all classified as CIN III. An example of this is shown in Figure 2(a). In the other 15 cases larger areas with unclear boundaries showed elevated DR values. An example of a more diffuse fluorescence image is given in Figure 2(b). In these cases the location with the highest DR never coincided with the location where a biopsy was taken. Figure 2White light images and corresponding fluorescence images of two cases histopathologically confirmed as CIN III. The locations where the biopsies were taken are indicated by a square, and the ellipse indicates the position of the portio in the fluorescence image. In (a) a clearly localized spot is visible in the fluorescence image and it corresponds to the location where the biopsy was taken. In (b) a more diffuse increase in the DR is observed. ![]() 3.4.Staging of CIN With DR Fluorescence ImagingFigure 3 shows the DR value averaged over a 16×16 pixel square located at the location at which the biopsy was taken versus the histopathological stage. Although some outliers are clearly present, there appears to be a significant correlation between the DR value and the histopathology [Spearman rank correlation, r=0.881, p<0.001 (confidence interval 0.7044–0.9552)]. 4.Discussion4.1.LocalizationFluorescence images were obtained of a total of 16 cervices. In 13 of these we have histopathological confirmation of intraepithelial neoplasia. In 4 (of these 13) a clearly localized lesion was visible in the fluorescence image at a location coinciding with the (previously determined) location of the biopsy. The biopsies from these sites were all classified as CIN III. In the other nine cervices the fluorescence images showed multiple hotspots of varying intensity or more diffuse elevation of the fluorescence DR. This suggests a more multifocal nature of the disease in these cases. 4.2.StagingThe strong correlation between the DR value and the histopathological classification shown in Figure 3 is quite striking. This might suggest that there is a relation between the amount of PpIX that accumulates and the stage of the disease. However, we believe that a different mechanism is behind this phenomenon. In the derivation of Eq. (1) a semi-infinite homogeneous medium with a homogeneous PpIX distribution is assumed. The clinical situation is obviously a lot more complex. In the case of topically applied ALA on a mucosal surface a PpIX containing layer may have a thickness of up to a few hundred micrometers of the order of, or less than, the penetration depth of the violet excitation light. Furthermore, an epithelial tumor originates from the boundary between the stromal and epithelial layers and then progresses towards the top surface, into the monitored tissue volume. This will increase the average porphyrin concentration in this volume. As a consequence, we must seriously doubt the validity of the assumption of a semi-infinite homogeneous distribution of PpIX. To gain more insight into the dependence of the DR on the layered clinical situation, we performed Monte Carlo simulations for which we defined a two-layered structure that represented the epithelial layer and an underlying stromal layer. Both layers were assumed to autofluoresce, and only the epithelial layer was assumed to produce PpIX fluorescence. Fluorescence emerging from the surface was calculated for both excitation and detection wavelengths and a DR value was calculated. By performing different simulations with various layer thicknesses we could assess the dependence of the DR on the layer thickness. The input parameters for the calculations were obtained from the literature and from personal measurements. The technique and the methods are described more in detail in the Appendix. The results of the simulations are depicted in Figure 4. In comparing these results to Figure 3 it is highly likely to link the stage of the disease to the thickness of the tumor. However, the representation of tumor progression by a perfectly smooth walled cube is far from a realistic situation. Moreover, the optical properties of cervical tissue are not known and the values used in the Monte Carlo simulation were, at best, a guess. However, the values and the geometrical assumption used for the Monte Carlo simulation were used to investigate a concept that may give insight into the correlation between the DR and the stage of the disease. The validity of this concept does not depend on the exact values of the input parameters as long as the relative relations are reasonable. 4.3.MethodologyTracing the location where the biopsy was taken on the fluorescence image was done by hand. Two different imaging devices were used and a topographical match between the two images was made based on visual clues and landmarks, like the portio and the edge of the cervix. Although a “double blind” approach was used in this procedure, it remains the “Achilles heel” of the study. This problem could very simply be avoided by using an integrated device thereby performing both photographic documentation and DR imaging through the same optics. Technically this should not be a major problem, and the preliminary results obtained in this study certainly warrant such a development. 5.ConclusionsDR fluorescence imaging of ALA induced PpIX of the cervix using our general purpose prototype appeared to be feasible. In four cases strongly localized fluorescent hotspots were observed at the location where clinically the disease was colposcopically visible. In the other cases the fluorescence showed a more diffuse multifocal image. The value of the DR determined at the site of biopsy correlated in a statistically significant way with the histopathologically determined stage of the disease. This suggests that noninvasive staging of CIN using this technique is feasible. We believe that the results of this study justify the development of a dedicated device that combines regular white light colposcopy with DR fluorescence imaging. AcknowledgmentsThis work was funded by the Dutch Technology Foundation, Grant No. AGN 443413, and the European Commission, Contract No. BMH4-CT 97-2260. AppendixFor the calculations, we adjusted a Monte Carlo program, based on the code of Keijzer et al.;25 and adapted by Lucassen et al.;26 The program uses a variance reduction method that is called survival weighting. The photons are transported through the medium with a certain weight. At each interaction, a fraction μa/(μa+μs) of the weight is deposited while the remaining fraction continues. The program was adjusted to enable fluorescence imaging simulation. First, the distribution of the excitation light is calculated using the source type and dimensions and the optical properties of the medium as input parameters. Light that is absorbed in the medium is scored in a (z, r) matrix that is used to assign weight to the fluorescence sources. A separate input file that contains the distribution of the fluorophores is used as a source file for calculation of the fluorescent light distribution. The fraction of photons that escapes the medium from the top layer within a certain escape angle is scored in a matrix. Relative fluorescence levels are determined for semi-infinite double layer geometries (representing the epithelial layer and the stromal layer) with embedded square geometries representing neoplastic areas. For the simulations, the thickness of the neoplastic area that originates at the boundary of the two layers was varied. The epithelial layer is first set to 0.5 mm4. The “tumor” progresses from the boundary between the two layers into the top layer [Figures 5(a)–5(d)]. Some simulations were also performed with an increase in the thickness of the total epithelial layer from 0.5 to 1.3 mm [Figures 5(d)–5(f)] according to the findings of Abdul-Karim et al.;27 To our knowledge, the optical properties of cervical tissue in the blue light range are not known. For the simulations of excitation light we have chosen an absorption coefficient and a scattering coefficient in the range of aorta tissue found by Keijzer et al.;28 Excitation optical properties: μa: 15 cm−1 and μs : 400 cm−1. The fluorescence optical properties were chosen to be in the range found by Hornung et al.;:4 layer 1: μa: 0.15 cm−1, μs: 90 cm−1, g: 0.9, n: 1.37, thickness: 500–1300 μm; layer 2: μa: 1 cm−1, μs : 150 cm−1, g: 0.9, n: 1.37 thickness: >5 cm. REFERENCES
M. F. Mitchell
,
W. N. Hittelman
,
W. K. Hong
,
R. Lotan
, and
D. Schottenfeld
,
“The natural history of cervical intraepithelial neoplasia: An argument for intermediate endpoint biomarkers,”
Cancer Epidemiol. Biomarkers Prev. , 3 619
–626
(1994). Google Scholar
L. G. Koss
,
“The Papanicolaou test for cervical cancer detection. A triumph and a tragedy,”
J. Am. Med. Assoc. , 261 737
–743
(1989). Google Scholar
R. J. Kurman
,
D. E. Henson
,
A. L. Herbst
,
K. L. Noller
, and
M. H. Schiffman
,
“Interim guidelines for management of abnormal cervical cytology. The 1992 National Cancer Institute Workshop,”
J. Am. Med. Assoc. , 271 1866
–1869
(1994). Google Scholar
R. Hornung
,
T. H. Pham
,
K. A. Keefe
,
M. W. Berns
,
Y. Tadir
, and
B. J. Tromberg
,
“Quantitative near-infrared spectroscopy of cervical dysplasia in vivo,”
Hum. Reprod. , 14 2908
–2916
(1999). Google Scholar
E. Hopman
,
P. Kenemans
, and
Th. Helmerhorst
,
“Positive predictive rate of colposcopic examination of the cervix uteri: An overview of literature,”
Obstet. Gynecol. Surv. , 53 97
–106
(1998). Google Scholar
J. Sellors
,
P. Nieminen
,
E. Vesterinen
, and
J. Paavonen
,
“Observer variability in the scoring of colpophotographs,”
Obstet. Gynecol. (N.Y.) , 76 1006
–1008
(1990). Google Scholar
S. M. Ismail
,
A. B. Colclough
,
J. S. Dinnen
,
D. Eakins
,
D. M. Evans
,
E. Gradwell
,
J. P. O’Sullivan
,
J. M. Summerell
, and
R. G. Newcombe
,
“Observer variation in histopathological diagnosis and grading of cervical intraepithelial neoplasia,”
Br. Med. J. , 298 707
–710
(1989). Google Scholar
E. Buxton
,
D. Luesley
,
M. Shafi
, and
M. Rollason
,
“Colposcopically directed punch biopsy: A potentially misleading investigation,”
Br. J. Obstet. Gynaecol. , 98 1273
–1276
(1991). Google Scholar
E. Hopman
,
F. Voorhorst
,
P. Kenemans
,
C. Meyer
, and
Th. Helmerhorst
,
“Observer agreement on interpreting colposcopic images of CIN,”
Gynecol. Oncol. , 58 206
–209
(1995). Google Scholar
J. R. Mourant
,
A. H. Hielscher
,
A. A. Eick
,
T. M. Johnson
, and
J. P. Freyer
,
“Evidence of intrinsic differences in the light scattering properties of tumorigenic and nontumorigenic cells,”
Cancer , 84 366
–374
(1998). Google Scholar
L. T. Perlemand
et al.;,
“Observation of periodic fine structure in reflectance from biological tissue: A new technique for measuring nuclear size distribution,”
Phys. Rev. Lett. , 80 627
–630
(1998). Google Scholar
G. Zonios
,
R. Cothren
,
J. M. Crawford
,
M. Fitzmaurice
,
R. Manoharan
,
J. van Dam
, and
M. S. Fels
,
“Spectral pathology,”
Ann. N.Y. Acad. Sci. , 838 108
–115
(1998). Google Scholar
G. Zonios
,
R. Cothren
,
J. T. Arendt
,
H. C. Wu
,
J. van Dam
,
J. M. Crawford
,
R. Manoharan
, and
M. S. Feld
,
“Morphological model of human colon tissue fluorescence,”
IEEE Trans. Biomed. Eng. , 43 113
–122
(1996). Google Scholar
M. F. Mitchell
,
S. B. Cantor
,
N. Ramanujam
,
G. Tortolero-Luna
, and
R. Richards-Kortum
,
“Fluorescence spectroscopy for diagnosis of squamous intraepithelial lesions of the cervix,”
Obstet. Gynecol. (N.Y.) , 93 462
–470
(1999). Google Scholar
A. Major
et al.;,
“In vivo fluorescence detection of ovarian cancer in the NuTu-19 epithelial ovarian cancer animal model using 5-aminolevulinic acid (ALA),”
Gynecol. Oncol. , 66 122
–132
(1996). Google Scholar
M. K. Fehr
,
P. Wyss
,
B. J. Tromberg
,
T. Krasieva
,
P. J. DiSaia
,
F. Lin
, and
Y. Tadir
,
“Selective photosensitiser localization in the human endometrium after intrauterine application of 5-aminolevulinic acid,”
Am. J. Obstet. Gynecol. , 175 1253
–1259
(1996). Google Scholar
J. Bedwell
,
A. J. MacRobert
,
P. Phillips
, and
S. G. Boan
,
“Fluorescence distribution and photodynamic effect of ALA-induced PpIX in the DMH rat colonic tumour model,”
Br. J. Cancer , 65 818
–824
(1992). Google Scholar
S. A. Pahernik
et al.;,
“Pharmacokinetics and selectivity of aminolevulinic acid-induced porphyrin synthesis in patients with cervical intra-epithelial neoplasia,”
Int. J. Cancer , 78 310
–314
(1998). Google Scholar
M. Hillemans
,
H. Weingandt
,
R. Baumgartner
,
J. Diebold
,
W. Xiang
, and
H. Stepp
,
“Photodetection of cervical intraepithelial neoplasia using 5-aminolevulinic acid induced porphyrin fluorescence,”
Cancer , 88 2275
–2282
(2000). Google Scholar
M. Sinaasappel
and
H. J. C. M. Sterenborg
,
“Quantification of the hematoporphyrin derivative by fluorescence measurement using dual-wavelength excitation and dual-wavelength detection,”
Appl. Opt. , 32 541
–548
(1993). Google Scholar
H. J. C. M. Sterenborg
,
A. E. Saarnak
,
R. G. J. Frank
, and
M. Motamedi
,
“Evaluation of spectroscopic correction techniques for in vivo fluorescence measurements on pigmented lesions,”
J. Photochem. Photobiol. , B35 159
–165
(1996). Google Scholar
A. Bogaards
,
M. C. G. Aalders
,
A. J. L. Jongen
,
E. Dekker
, and
H. J. C. M. Sterenborg
, Rev. Sci. Instrum. , 72 3956
–3961
(2001). Google Scholar
M. Keijzer
,
J. W. Pickering
,
S. A. Prahl
,
A. J. Welch
,
“Light distributions in artery tissue: Monte Carlo simulations for finite-diameter laser beams,”
Lasers Surg. Med. , 9 148
–154
(1989). Google Scholar
G. W. Lucassen
,
W. Verkruysse
,
M. Keijzer
, and
M. J. van Gemert
,
“Light distributions in a port wine stain model containing multiple cylindrical and curved blood vessels,”
Lasers Surg. Med. , 18 345
–357
(1996). Google Scholar
F. W. Abdul-Karim
,
Y. S. Fu
,
J. W. Reagan
, and
W. B. Wentz
,
“Morphometric study of intraepithelial neoplasia of the uterine cervix,”
Obstet. Gynecol. (N.Y.) , 60 210
–214
(1982). Google Scholar
M. Keijzer
,
R. R. Richards-Kortum
,
S. L. Jacques
, and
M. S. Feld
,
“Fluorescence spectroscopy of turbid media: Autofluorescence of the human aorta,”
Appl. Opt. , 28 4286
–4292
(1989). Google Scholar
|
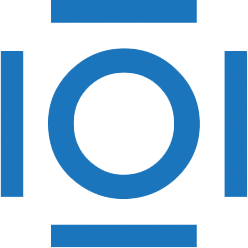
CITATIONS
Cited by 32 scholarly publications.
Luminescence
Biopsy
Cervix
Tissues
Monte Carlo methods
Tumors
Prototyping