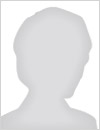
This will count as one of your downloads.
You will have access to both the presentation and article (if available).
The Coronagraph Instrument (CGI) will be required to operate with low signal flux for long integration times, demanding all noise sources are kept to a minimum. The Electron Multiplication (EM)-CCD has been baselined for both the imaging and spectrograph cameras due its ability to operate with sub-electron effective read noise values with appropriate multiplication gain setting. The presence of other noise sources, however, such as thermal dark signal and Clock Induced Charge (CIC), need to be characterized and mitigated. In addition, operation within a space environment will subject the device to radiation damage that will degrade the Charge Transfer Effciency (CTE) of the device throughout the mission lifetime. Irradiation at the nominal instrument operating temperature has the potential to provide the best estimate of performance degradation that will be experienced in-flight, since the final population of silicon defects has been shown to be dependent upon the temperature at which the sensor is irradiated.
Here we present initial findings from pre- and post- cryogenic irradiation testing of the e2v CCD201-20 BI EMCCD sensor, baselined for the WFIRST coronagraph instrument. The motivation for irradiation at cryogenic temperatures is discussed with reference to previous investigations of a similar nature. The results are presented in context with those from a previous room temperature irradiation investigation that was performed on a CCD201-20 operated under the same conditions. A key conclusion is that the measured performance degradation for a given proton fluence is seen to measurably differ for the cryogenic case compared to the room temperature equivalent for the conditions of this study.
Throughout the lifetime of a space-based mission the detector will be bombarded by high-energy particles and gamma rays. As time progresses, the radiation will damage the detectors, causing the Charge Transfer Efficiency (CTE) to decrease due to the creation of defects or “traps” in the silicon lattice of the detector. The defects create additional energy levels between the valence and conduction band in the silicon of the detector. Electrons or holes (for n-channel or p-channel devices respectively) that pass over the defect sites may be trapped. The trapped electrons or holes will later be emitted from the traps, subject to an emission-time constant related to the energy level of the associated defect. The capture and emission of charge from the signal leads to a characteristic trailing or “smearing” of images that must be corrected to enable the science goals of a mission to be met.
Over the past few years, great strides have been taken in the development of the pocket-pumping (or strictly-speaking “trap pumping”) technique. This technique not only allows individual defects (or traps) within the device to be located to the sub-pixel level, but it enables the investigation of the trap parameters such as the emission time constant to new levels of accuracy. Recent publications have shown the power of this technique in characterising a variety of different defects in both n- and p-channel devices and the potential for use in correction techniques, however, we are now exploring not only the trap locations and properties but the life cycle of these traps through time after irradiation. In orbit, most devices will be operating cold to suppress dark current and the devices are therefore cold whilst undergoing damage from the radiation environment. The mobility of defects varies as a function of temperature such that the mix of defects present following a cryogenic irradiation may vary significantly from that found following a room temperature irradiation or after annealing. It is therefore essential to study the trap formation and migration in orbit-like conditions and over longer timescales.
In this paper we present a selection of the latest methods and results in the trap pumping of n- and p-channel devices and demonstrate how this technique now allows us to map radiation-induced defects in CCDs through both space and time.
View contact details
No SPIE Account? Create one